teaser
K Chitkara
MRCP
Clinical Research Fellow
E:[email protected]
K Hogrefe
MRCP
Specialist Registrar
M Vasa-Nicotera
MD
Clinical Research Fellow
AH Gershlick
FRCP
Consultant Cardiologist
Department of Cardiology
Glenfield Hospital
Leicester
UK
Charles Dotter, known as the father of interventional radiology, introduced transluminal angioplasty in 1964.(1) In 1977 Gruentzig et al reported the first use of percutaneous transluminal coronary angioplasty (PTCA) as a minimally invasive alternative to bypass surgery.(2) The first human implantation of a coronary stent was performed by Jacques Puel (France) in 1986. The idea of a balloon-mounted stent for simultaneous dilatation and stent delivery was introduced by Palmaz and colleagues.(3) The advent of stents and their widespread use has undoubtedly provided not only a huge improvement in the success of interventional procedures, but has also brought a plethora of new complications, such as in-stent restenosis (ISR) and thrombosis.
Coronary stent placement is the most commonly employed technique for percutaneous treatment of atherosclerotic heart disease. It accounts for about 75% of the procedures performed worldwide,(4) and has revolutionised the practice of interventional cardiology over the past five years. In comparison to balloon dilatation, the use of stents has reduced the rate of restenosis, but ISR,(5–7) due to neointimal hyperplasia,(8,9) remains the major-long term limitation of this technique, occurring in 15–20% of cases. Moreover, in the Stent Restenosis Study (STRESS),(10) certain subsets of patients, such as those with diabetes mellitus, smaller vessels and complex lesions, reached an unacceptable high rate of 30–50% restenosis.
ISR is primarily due to neointimal hyperplasia. Following vascular injury, platelets, damaged endothelial cells, smooth muscle cells (SMCs) and macrophages secrete chemotactic factors, cytokines and growth factors, which trigger a complex array of events that induces cellular migration and proliferation,(11–13) leading ultimately to neointimal formation. This vasculoproliferative pathway involves the progression of SMCs through various phases of the cell cycle.
Growth factors or mechanical trauma stimulate vascular SMCs out of their quiescent G(0) phase. They enter the G(1) phase, where progression towards S phase is controlled by assembly and phosphorylation of a complex made out of a growth factor receptor (cyclin D or E) and cyclin-dependent kinase (CDK). Endogenous inhibitors (CKI) of these complexes come from the CIP/KIP and INK4 families. The balance of cyclin/CDK and CKI determines the progression of the cell through the G(1) phase. The complete sequence leading to the transition from G(1) to S phase is not fully understood, but once the cell has completed this step it is set to complete the whole cell cycle. There is some evidence that SMC migration might also be regulated through the cell cycle.(14,15)
Various systemic drugs with different pharmacological properties (eg, antiplatelets, antithrombotics, anti-inflammatories and antiproliferatives) have been tested in humans and found to be ineffective for the prevention of ISR,(16) including polymers (some of which provoked severe reactions),(17) silicon, gold, and heparin,(18–20) despite showing promise in experimental models.(15,21–23)
It is illogical to use systemic doses of a medication when only a localised area of an artery requires treatment, and exposes patients to side-effects from high doses of systemic drugs. The focus in interventional cardiology has shifted to local drug delivery using stents, since they can serve as a reservoir for local drug administration.
Stent loading techniques
The initial bare metal stent was thought to cause an inflammatory or foreign body response that fuelled the restenosis process. This led to modifications in stent design including different coatings to render the stent “tissue friendly”. Some of these polymer coatings were able to absorb additional drugs like a sponge,(24) and a new research area was pioneered.
Stent-based drug delivery is a complex modality that depends upon the tissue, drug and device. The stent struts cover 10–15% of the vessel area, limiting the surface area available to adsorb and elute drug from the stent. Various loading methods are available such as dipping, spraying, surface coating and mixing the drug with a noninflammatory polymer as a vehicle to elute the drug in a controlled release manner.(25)
Passive absorption into the stent coat is limited by the total amount of drug that can be loaded onto the stent. Elution is dependent on the chemical characteristics of the agent and follows a bi-exponential pattern, with a rapid initial elution followed by a more sustained elution phase lasting up to a few weeks (see Figure 1).
[[HPE10_fig1_48]]
Future developments in this field include multi-layer coatings. The agent is mixed with the polymer first and then applied onto the stent in layers. It can then be covered with a protective final layer to prevent initial rapid loss of the agent and lessen contact of the polymer with the vessel wall, thus making it less inflammatory. The drug will be freed due to hydrolysis of the polymer. For example, the new Conor med-stent has reservoirs containing admixture of paclitaxel in PLGA, maintaining equal drug loading in the wells.
First generation drug-eluting stents
The search for the right agent identified many promising candidates as a result of positive results in cell or tissue culture experiments. Some agents have also been shown to reduce restenosis in vivo in animal experiments. However, only a few have made it to clinical trials (Table 1).
[[HPE10_table1_48]]
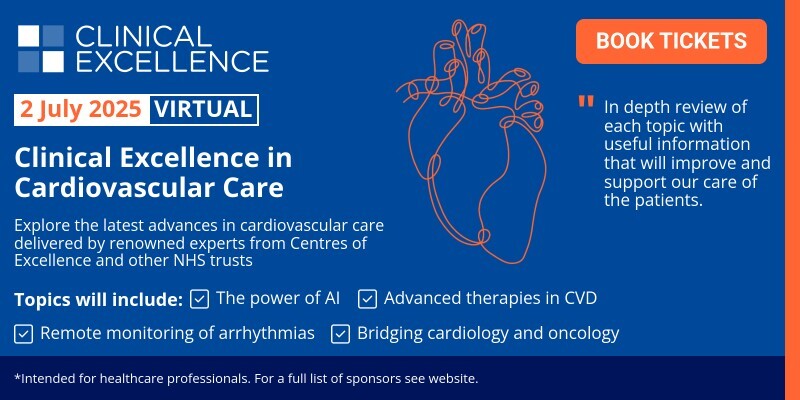
Rapamycin (sirolimus)
Rapamycin is a naturally-occurring macrolide antibiotic produced by Streptomyces hygroscopicus, and was originally found on Easter Island. The name is derived from Rapa Nui, the native name for Easter Island. Rapamycin is a hydrophobic synthetic drug, approved by the FDA in 1997 as an oral immunosuppressive agent to prevent organ transplant rejection. It is a macrocylic lactone that interacts with cell-cycle regulating proteins and inhibits cell division between phases G(1) and S(1), thereby inhibiting cellular proliferation (Figure 2). Its action is mediated by binding to an intracellular receptor – the complex formed inhibits the activity of a specific kinase, which prevents mitogen-induced downregulation of p27 (KIP1) by an unknown mechanism. (26)
[[HPE10_fig2_49]]
Being a lipophilic drug, rapamycin increases in concentration in the vessel wall and when the drug is mixed with polymer, it allows control over release kinetics. Its pharmacological advantages are: a long half life in tissues, diffuses across vessel wall, is cytostatic, not cytotoxic, and allows completion of re-endothelialisation.
Concerns about potential late complications such as thrombosis due to delayed re-endothelialisation, aneurysm, late restenosis, late occlusion or rebound hyperplasia have not been confirmed during up to three years follow-up in the FIM Trial (presented at the CRF Symposium of the American College of Cardiology conference 2003) (see Table 2).
[[HPE10_table2_49]]
The ORBIT study is examining the use of oral rapamycin to inhibit restenosis – early reports show low rates of restenosis and late loss. Adverse events include diarrhoea, oral mucocutaneous symptoms, skin rash, fever, gum infection, insomnia and headache. It is not clear whether these effects were related to treatment. It is unlikely that this strategy will be taken up. Similarly, oral everolimus (a derivative of rapamycin) was associated with significant neointimal healing in the rabbit iliac artery.(32)
Paclitaxel
In 1963 an extract from the bark of Taxus brevifolia was found to have cytotoxic activity against tumours by its effect on microtubular function and found to be highly lipophilic. Microtubules are formed by polymers of tubulin. Their principal function is in the formation of the mitotic spindle during cellular division.(31) They help to maintain cell shape and are intimately related to the functions of intracellular transport, signalling, protein secretion and motility.(32)
Paclitaxel specifically inhibits microtubules by inhibiting their depolymerisation resulting in an inhibition of cellular replication at the G(0)/G(1)/M phases (Figure 2).(33–35) This means paclitaxel blocks a cell’s ability to break down the mitotic spindle during mitosis, preventing it dividing into daughter cells. The paclitaxel derivative QP2 (7-hexanoyl taxol) has similar properties and has also been tested for the prevention of restenosis. In experimental models of restenosis, local administration of paclitaxel led to a significant neointimal reduction,(36,37) with a clear dose-dependent effect. Table 3 lists the results of some of the trials using paclitaxel-coated stents.
[[HPE10_table3_50]]
Newer agents
Tacrolimus
Tacrolimus, an immunosuppressant used in heart and renal transplant patients, is a derivative of soil fungus (Streptomyces tsukubaensis). Tacrolimus is a hydrophobic immunosuppressive agent; it binds to the FKBP12 protein and inhibits the release of proinflammatory cytokines and activation of T cells. Six months data from PRESENT I, PRESENT II and EVIDENT trials of tacrolimus-coated stents were presented at the ACC CRF Symposium 2003. Angiographic restenosis rate was 19%, 21% and 27% respectively, but with small numbers in pilot trials.
Everolimus
Everolimus has been shown to inhibit proliferation of haematopoietic and nonhaematopoietic cells. It is a potent antirestenotic agent given orally or via a drug-eluting stent. At ACC March 2003, six months data (FUTURE I trial) in 42 patients (low risk, not diabetic and short lesions) showed mirror image results of RAVEL; 0% binary stenosis vs 9.1% in control, p=ns.
ABT 578
ABT 578, methylrapamycin, a synthetic analogue of sirolimus, is an immunosuppressant that inhibits SMC proliferation by blocking the function of the cell cycle regulatory protein, mTOR. It has a cytostatic action on cells by blocking entry into the S phase of the cell cycle. ABT 578 PC coated stent study showed encouraging results in pigs (n=20), with a 46% reduction in neointimal thickness (p<0.001).(42) Enrolment has started in the ENDEAVOR II study to implant ABT 578 PC-coated stent in humans for the treatment of single de novo lesions in native coronary arteries with a diameter of 3.0–3.5mm.
Conclusion
Patients in drug-eluting stent (DES) trials need to be followed up long term, to demonstrate not only durability of the initial promising results, but also efficacy with small vessels, long lesions, bifurcations, left main stem diseases and chronic occlusions. Currently, only three DES have received the CE mark and are available in Europe (Cordis’ CYPHER, sirolimus-eluting stent; Boston Scientific’s TAXUS, paclitaxel-eluting stent; Abbott’s DEXAMET, dexamethasone- eluting stent system). In future, we expect to see many other drug coated stents.
The cost of these stents is still a major issue. The current cost of a DES is between £900 and £1,500 (£1=euro 1.41) and the total cost increases when the patient has more than one lesion that requires PCI and possible multiple DES. A recent independent economic analysis of the SIRIUS Trial showed that for every 100 patients treated with CYPHER Stents, there were 19 fewer revascularisations and 25 fewer hospital admissions.
There are still issues about re-emergence of neointimal hyperplasia when the drug is completely released from the stent, and there have been a few unproven cases of subacute thrombosis secondary to use of sirolimus-coated stents.
There could be a future beyond DES, for example; CD-34 antibody coated stents to capture endothelial progenitor cells in the blood stream and promote endothelialisation (First in Man BIO Brazil study), and systemic injection of bisphosphonates to inhibit monocytes/macrophages. More research is required into homologous coating of stents, and design and biocompatibility of stents. There may be a need for an agent with dual antithrombotic and antirestenotic properties, or double drug loading onto a stent as there are issues of thrombosis within the stent. Eptifibatide, an antithrombotic drug licensed for unstable angina, recently showed in-vitro inhibition of cell proliferation and migration in response to various chemotactic factors, thus acting as an antirestenotic agent.(43)
References
- Dotter CT, Judkins MP. Radiology 1989;172:904-20.
- Senning AR, et al. N Engl J Med 1979;301:61-8.
- Palmaz JC, Sibbitt RR. Radiology 1985;156:73-7.
- Pocock SJ, Henderson RA. Lancet 1995; 346:1184-9.
- Fischman DL, Leon MB. N Engl J Med 1994;331: 496-501.
- Versaci F, et al. N Engl J Med 1997;336:817-22.
- Serruys PW, et al. N Engl J Med 1994;331:489-95.
- Hoffmann R, Mintz GS. Circulation 1996;94:1247-54.
- Virmani R, et al. Curr Opin Lipidol 1999;10:499-506.
- Fischman DL, Leon MB. N Engl J Med 1994;331: 496-501.
- Farb A, et al. Circulation 1999;99:44-52.
- Kornwski R, et al. J Am Coll Cardiol 1998;31:224-30.
- de Feyter PJ, et al. Curr Interv Cardiol Rep 2000;2:326-31.
- Hong MK, et al. Circulation 2003;107:517-20.
- Sun J, et al. Circulation 2001;103:2967-72.
- Ahn YK, et al. Catheterization & Cardiovascular Interventions 1999;48:324-30.
- van der Giessen WJ, Lincoff AM. Circulation 1996;94:1690-7.
- Malik N, et al. J Invasive Cardiol 2001;13:193-201.
- Edelman ER. Circulation 2001;103:429-34.
- Park S-JM. Am J Cardiol 2002; 89:872-5.
- Wohrle JA,.et al. Eur Heart J 2001;22:1808-16.
- Vom Dahl JM, et al. Am J Cardiology 2002;89:801-5.
- Takagi T, et al. J Am Coll Cardiol 2000;36:1529-35.
- Aggarwal RK, et al. Circulation 1996;94:3311-7.
- Finkelstein A, et al. Circulation 2003; 107(5):777-84.
- Marx SO, Marks AR. Circulation 2001;104:852-5.
- Sousa JE, et al. Circulation 2001; 104:2007-11.
- Kozuma K, et al. Eur Heart J 2001;22:44-6.
- Colombo AM, Orlic DM. Circulation 2003;107:2178-80.
- Sousa JE, et al. Circulation 2001;103:192-5.
- Jeffery W Moses, et al. TCT Meeting 2002.
- Farb A, et al. Circulation 2002;106:2379-84.
- Schiff PB, et al. Proc Natl Acad Sci 1980;77:1561-5.
- Jordan MA, et al. Proc Natl Acad Sci 1993;90:9552-6.
- Honda Y, et al. Circulation 2001; 104:380-3.
- Hong MK, et al. Coron Artery Dis 2001;12:513-5.
- Heldman AW, Cheng L. Circulation 2001;2289-95.
- Grube E, et al. Circulation 2003;107:38-42.
- Colombo A. American College of Cardiology Scientific Sessions 2003.
- Tanabe K, et al. Circulation 2003;107:559-64.
- Gershlick AH. British Cardiac Society meeting 2002.
- Carter AJ. J Am Coll Cardiol 2003;41:6A.
- Chitkara K, et al. TCT Meeting 2003.