Rashmi R Shah
Senior
Medical Officer
Medicines
Control Agency
London, UK
E:[email protected]
When the structure of a drug includes an asymmetric (also known as chiral) centre, it can exist as two mirror images that cannot be superimposed three-dimensionally, known as enantiomers (see Figure 1). Apart from differences in their ability to rotate plane-polarised light, enantiomers of a drug may also differ in their biological activities.
[[HPE06_fig1_25]]
The enzymes that metabolise drugs and the pharmacological targets of these drugs are built from chirally pure building blocks, so it is not surprising that there may be differences between the two enantiomers in their pharmacokinetics and/or pharmacodynamics. Since the major drug metabolising enzymes, such as CYP2D6, CYP2C9 and CYP2C19, display genetic polymorphism within a population, stereoselectivity in drug metabolism is intricately linked with pharmacogenetics.
The significance of stereoselectivity in drug action was known as long ago as 1926 with the knowledge that the biological activity of atropine resided in only one stereoisomer.(1) The enantiomer with the higher affinity for the primary pharmacological target is known as the eutomer, while the other is known as the distomer. Although there were a few synthetic drugs available in chirally pure form, such as L-dopa and D-penicillamine (see later), the lack of suitable methods for either the large-scale preparation of pure enantiomers or for stereoselective analysis meant that the majority of stereochemically pure drugs on the market were of only natural origin. Consequently, the discussion on whether enantiomers of a chirally active drug should be developed was largely an academic one and, historically, synthetic chiral drugs were mainly presented as the racemate.
Drug development now increasingly involves pure enantiomers, either de novo or of previously marketed racemic drugs (“chiral switch”). A number of factors have contributed to this, including advances in enantioselective analytical techniques that have resulted in a greater appreciation of enantioselective pharmacology and its impact on the risk/benefit of a drug. Technological advances have also made it possible to produce pure enantiomers on a commercial scale.
This article focuses on the pharmacological principles and the regulatory framework that should guide the development of single enantiomers. For a more detailed discussion on stereochemistry in drug development, the reader is referred to other reviews.(2–4)
Principles of chiral pharmacology in drug development
Examination of the pharmacological properties of enantiomers reveals three possibilities:
- Both enantiomers may have similar activities.
- One enantiomer is pharmacologically active, while the other is inactive.
- Both enantiomers have quite different activities or have different potency for a given activity.
There are no clinical safety or efficacy implications arising from the first two possibilities. The third possibility, however, offers exciting opportunities for improving the clinical risk/benefit of the drugs concerned. Not surprisingly, this scenario has attracted considerable regulatory, academic and commercial interest.
Developing racemic mixtures
Reasons for developing a racemic mixture could include the facts that:
- Both enantiomers have similar pharmacological activities.
- Both enantiomers have different but therapeutically complementary activities.
- There is substantial chiral interconversion in vivo.
- The two enantiomers produce the same achiral active metabolite.
- The pharmacokinetic profile of the drug is nonstereoselective.
Developing single-enantiomer drugs
The main reasons for developing a single enantiomer include:
- One of the enantiomers is highly potent and there is hardly any chiral interconversion; this allows reduction of daily dose and consequently the “metabolic load”.
- The secondary pharmacology of the drug, responsible for toxic effects, resides predominantly in one enantiomer.
- The pharmacokinetics are enantioselective; this allows the choice of the isomer with long half-life in order to decrease dosing frequency or of the isomer with shorter half-life in order to diminish the risk of accumulation in susceptible population (eg, the elderly), or if overdose with the drug is a distinct clinical possibility.
- There is great interindividual variability that could be eliminated by developing only the enantiomer that is not subject to polymorphic metabolism.
- Therapeutically “inactive” enantiomer alters in a detrimental manner the pharmacokinetics (or pharmacodynamics) of the therapeutically active enantiomer.
- Notwithstanding the slight loss in potency, one of the enantiomers has the required “site-specificity”.
- The inhibition of drug-metabolising CYP isoforms by the two enantiomers is enantio-selective; this could be exploited by eliminating the interacting enantiomer to improve the drug–drug interaction profile of the enantiomer selected for development if drug interactions are likely to prove to be a major clinical problem with the racemic mixture.
The complex decision of whether to develop a racemate or a single enantiomer of chirally active drugs is left to the sponsors. This decision, however, requires justification and depends on detailed information on stereoselectivity in drug metabolism, renal clearance, pharmacodynamics of the parent drug and its metabolites, and on pharmacogenetic influences.
Regulatory framework for chirality in drug development
Regulatory control of chiral drugs was first considered by Sweden in the early 1980s. Arising from a greater appreciation of enantioselective pharmacology on the risk/benefit ratio of a drug, most regulatory authorities have now introduced guidance notes on the development of chiral drugs. They have all adopted essentially the same attitude, with slight differences in emphasis. The regulation of chiral drugs is pragmatic, based on scientific data relating to quality, safety, efficacy and risk/benefit.
As early as 1987, the US Food and Drug Administration (FDA) required documentation concerning the pharmacological, preclinical and clinical properties of enantiomers in the new drug applications. In January 1992, the FDA formally published a policy statement for the development of new stereoisomeric drugs, with amendments made in January 1997. The European Union’s guidance note on “Investigation of chiral active substances” for drugs that may exist as optical isomers (CPMP/III/3501/ 91) came into force in April 1994. The Canadian Health Protection Branch also adopted a guideline in 1994, which was updated in 1996 and again in February 2000. All these guidelines set out requirements for studies to justify the chosen strategy in the areas corresponding to the three technical parts (pharmaceutical, preclinical and clinical) of the dossier accompanying the application.
The Japanese Ministry of Health and Welfare (MHW) has not issued specific guidance on the development of chiral drugs, but has nonetheless responded to the “enantiomer- versus-racemate” scientific debate, and there is a considerable degree of concordance with the regulatory principles established elsewhere.
Development of single enantiomers
Not surprisingly, as a result of guidance notes issued by regulatory authorities, the proportion of synthetic new drugs marketed as single enantiomers has increased significantly over the last decade.(4)
When there are doubts or the sponsor decides to deviate from the guidelines, they are encouraged to discuss the issue as early as possible with the appropriate regulatory authority. Discussions between the sponsor and the regulatory authority are the norm in Japan, the EU and the US. The FDA is also happy to discuss any cases where questions exist regarding the definition of “significant toxicity” in the context of the possible role of an individual enantiomer in an unexpected preclinical toxicity finding.
In the EU, discussions are possible not only with individual Member States, but also with the Committee for Proprietary Medicinal Products to obtain pan-European formal scientific advice.
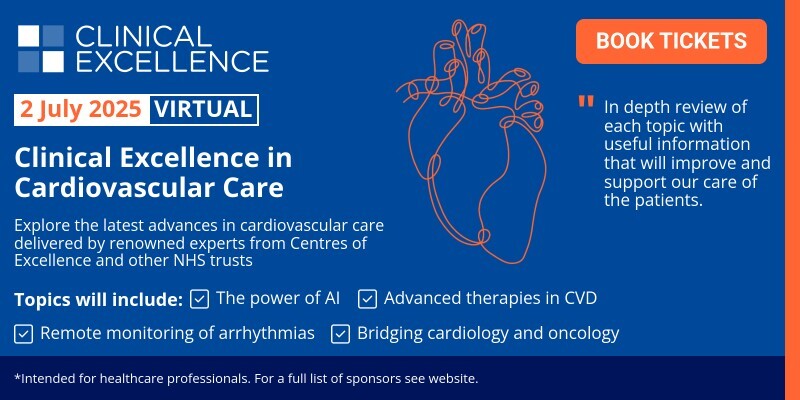
The requirements of data when contemplating a chiral switch may be briefly summarised as follows.
In terms of the pharmaceutical (quality) data required, the manufacture of a pure enantiomer requires a complete documentation just as for any new chemical entity. The enantiomer not intended for marketing may be conceptually treated as an impurity. Issues requiring close attention are the identity and the stereoisomeric purity of the active ingredient from early synthesis, to specifications of the finished product with respect to its composition, stability and proposed shelf-life.
In terms of preclinical and clinical data, as far as is applicable to the enantiomer, data on the corresponding racemate may be used in order to reduce the amount of new studies. Suitable “bridging” studies, determined on a case-by-case basis, should be carried out to link the complete racemate data to the incomplete data on the selected enantiomer. The way the racemate data are used should be explained and justified. When deciding on the extent of bridging studies, it is important to compare the pharmacodynamic and pharmacokinetic profiles of the selected enantiomer with that of the racemate. Evaluation should include the extent of chiral interconversion. In addition, it may be addition to study the other enantiomer to correct for interactions. If unexpected results are found preclinically, further studies on a case-by-case basis will be necessary. In the worst case, the enantiomer will need to be fully investigated.
The principles and requirements for clinical studies are identical to those for preclinical studies. Clinically, in principle the usual pharmacotherapeutic (efficacy) studies should all be carried out. However, with preclinical data on the racemate and the selected enantiomer as well as the therapeutic trials with the racemate, it may be possible to extrapolate data to the single enantiomer being developed. The clinical expert report should provide additional arguments to support extrapolation from one indication study with the single enantiomer to other indications.
Recent and potential chiral switches
Chiral switching represents one of the most common scenarios today. Examples of chiral switches, either potential or accomplished, include the development of (S)-bupivacaine, (–)-cetirizine, (S)-doxazosin, (+)-fenfluramine, (R)-fluoxetine, (S)-fluoxetine (for migraine), (R,R)-formoterol, (S)-ibuprofen, (S)-ketoprofen, (S)-ketamine, (R,R)-labetalol, (R)-lansoprazole, (S)-ofloxacin, (S)-oxybutynin, (R)-salbutamol, (R)-sibutramine and (S)-amlodipine among many others.(5) Most recently, chiral switching of the most widely used drugs has resulted in approval of esomeprazole (Nexium; AstraZeneca) and escitalopram (Cipralex; Lundbeck), single enantiomers of
the previously marketed racemic omeprazole and citalopram respectively.
All proton pump inhibitors (PPIs) exist as two enantiomers because of the presence of an asymmetric sulphinyl group. Parent PPIs are inactive prodrugs. Following accumulation in the acidic space, these parent compounds are converted to achiral thiophilic sulphenamide, which inactivates the H(+)/K(+)-ATPase pump, resulting in reduced gastric acid secretion. Since both enantiomers are transformed into the sulphenamide at the same rate, both parent enantiomers of PPIs are “equipotent”. The efficacy of these drugs correlates with the area under their plasma concentration–time curve (AUC). The metabolic elimination, however, is stereoselective for one of the enantiomers, principally mediated by the polymorphic CYP2C19. Thus administration of a racemate results in wide interindividual variability. This variability can be reduced, with a more predictable efficacy, by administering only the enantiomer that is less subject to polymorphic metabolism.
With regard to poor metabolisers (PM) and extensive metabolisers (EM) of CYP2C19, the ratios of AUC for PM/EM are 7.5 for (+)-(R)-omeprazole and 3.1 for (–)-(S)- omeprazole.(6) (–)-(S)-omeprazole is now approved and marketed as esomeprazole.(7) The usual dosage of esomeprazole for most indications is 20mg once daily, which is either the same or, in some indications, slightly higher than that of racemic omeprazole, bearing in mind that (+)-(R)-omeprazole is also active. However, at equimolar dose, the exposure is up to 70% higher following administration of (–)-(S)-omeprazole than following racemic omeprazole. Compared with the racemic drug, the recommended dose of (–)-(S)-omeprazole provides greater and more prolonged hypochlorhydria and this may have long-term safety implications.(8)
Following a suicidal overdose, citalopram has been reported to cause sudden death.(9) The pharmacological activity of citalopram resides in the (+)-(S)-enantiomer. Citalopram is also metabolised principally by CYP2C19. For (+)-(S)-citalopram and (–)-(R)-citalopram, the mean serum half-lives average 35 and 47 hours respectively.(10) Citalopram is metabolised to its two main metabolites, demethylcitalopram (DCT) and didemethylcitalopram (DDCT). Although DDCT is normally a minor metabolite in man, it may accumulate following an overdose. In-vitro studies show that DDCT is cardiotoxic and prolongs the QT interval.(9) Recent studies have shown that QT interval prolongation by citalopram is probably almost exclusively attributable to (–)-(R)-DDCT. Clinically, (+)-(S)-citalopram 10mg/daily was shown to have a superior and an earlier onset of therapeutic effect when compared with racemic citalopram 20mg/daily.(11) (+)-(S)-citalopram was recently approved as escitalopram tablets. The usual dosage of racemic citalopram for depression is 20mg daily, while that of escitalopram is 10mg once daily. Whether or not escitalopram is superior to the racemic drug in terms of its cardiac safety remains to be seen.
Esomeprazole and escitalopram represent two very different strategies in chiral switching; while the former was developed as a completely new drug, the latter relied on a bridging studies approach.
Mefloquine is a chiral antimalarial agent effective against chloroquine-resistant Plasmodium falciparum. The clinical use of racemic mefloquine is associated with serious neuropsychiatric side-effects that are dose-related but not plasma concentration-related. The development of (–)-mefloquine could prove a useful switch, providing this enantiomer is shown to be as effective as the racemic drug. In-vitro data suggest that (–)-mefloquine has antimalarial activity.(12) Following administration of racemic mefloquine, the plasma concentrations and AUC of the (–)-enantiomer are significantly higher than those of (+)-mefloquine.(13) It has been shown that (+)-mefloquine has higher brain penetration.(14) Could this explain the neuropsychiatric side-effects of racemic mefloquine? The possibility that (–)-mefloquine may have a superior risk/benefit ratio is worth investigating. This switch would be comparable to the introduction in 1961 of (+)-ethambutol in the treatment of tuberculosis.(15) With regard to optic neuritis, the risk/benefit ratio of this isomer was far superior to that of (–)-ethambutol. Another drug meriting investigation of chiral switch to a single enantiomer on safety grounds is halofantrine, also for malaria.(16)
Racemic terodiline was withdrawn from the market in 1991 because of its proarrhythmic risk. Chiral switch to (–)-(S)-terodiline could have been investigated since the proarrhythmic potential resided in (+)-(R)-terodiline.(17,18) Instead, the sponsor developed de novo a structural analogue as a chirally pure enantiomer, (+)-(R)-tolterodine.
When there is sufficient evidence of stereoselective toxicity, the de-novo development of single enantiomers is easy to justify. There are a few older drugs already developed as single enantiomers. The antipodes of L-dopa and D-penicillamine were associated with toxicity – D-dopa with granulocytopenia(19) and L-penicillamine with serious nephrotoxicity.(20) Other familiar but less well-appreciated examples of stereochemically pure drugs in clinical use include (+)-methorphan, which is an over-the-counter antitussive, while (–)-methorphan is a controlled narcotic, and (+)-alpha-propoxyphene, which is a potent analgesic, while (–)-alpha-propoxyphene is an active antitussive.
Chiral switching is not without unforeseen risks. Although the benefits, if any, are often not obvious, there is little doubt when safety is concerned. Examples of chiral switches that have run into problems include (+)-fenfluramine, which was withdrawn because of cardiac valvulopathy, and (R,R)-labetalol, which was withdrawn because of hepatotoxicity. The development of (R)-fluoxetine was terminated because of potential risk of QT interval prolongation.
Conclusion
The decision to switch to a single enantiomer should be based on sound pharmacological principles. Unfortunately, however, the decisions are often commercially driven and based on unsubstantiated assumptions of a therapeutic benefit over the corresponding racemate. When a chiral switch is warranted because of an emerging postmarketing safety concern, the sponsor often loses interest in favour of developing a new structural analogue.
Despite their short-term advantages, commercially driven decisions for chiral switches are likely to be counterproductive in the long term, especially if the more expensive single enantiomer is soon found to offer no tangible clinical advantage over the well- established racemic product.
The views expressed in this paper are those of the author and do not necessarily represent the views or the opinions of the Medicines Control Agency, other regulatory authorities or any of their advisory committees.
References
- Cushny AR. Biological relations of optically isomeric substances. London: Ballière, Tindall and Cox; 1926.
- Shah RR, et al. Adverse Drug React Toxicol Rev 1998;17:145-90.
- Shah RR. Improving clinical risk/benefit through stereochemistry. In: Eichelbaum M, et al, editors. Handbook of experimental pharmacology. Heidelberg: Springer-Verlag; 2002. p. 401-32.
- Shah RR, Branch SK. Regulatory requirements for the development of chirally active drugs. In: Eichelbaum M, et al, editors. Handbook of experimental pharmacology. Heidelberg: Springer-Verlag; 2002. p. 379-99.
- Tucker GT. Chiral switches. Lancet 1999;355:1085-7.
- Tybring G, et al. Clin Pharmacol Ther 1997;62:129-37.
- Spencer CM, Faulds D. Drugs 2000;60:321-9.
- Daniels IR. Lancet 2001;357:1290-1.
- Öström M, et al. Lancet 1996;348: 339-40.
- Sidhu J, et al. Chirality 1997;9:686-92.
- Montgomery SA, et al. Pharmacol Toxicol 2001;88:282-6.
- Karle JM, et al. Exp Parasitol 1993;76:345-51.
- Gimenez F, et al. J Pharm Sci 1994;83:824-7.
- Pham YT, et al. Int J Clin Pharmacol Ther 1999;37:58-61. [Erratum in Int J Clin Pharmacol Ther 1999;37:316.]
- Blessington B. Ethambutol and tuberculosis, a neglected and confused chiral puzzle. In: Aboul-Enein HY, Wainer IW, editors. The impact of stereochemistry on drug development and use. 1st ed. New York: John Wiley & Sons; 1997. p. 235-61.
- Wesche DL, et al. Clin Pharmacol Ther 2000;67:521-9.
- Hartigan-Go K, et al. Clin Pharmacol Ther 1996;60:89-98.
- Shah RR. Withdrawal of terodiline: a tale of two toxicities. In: Mann R, Andrews E, editors. Pharmacovigilance. 1st ed. Chichester (UK): John Wiley & Sons; 2002. p. 135-54.
- Cotzias GC, et al. N Engl J Med 1969;280:337-45.
- Williams KM. Pharmacol Ther 1990;47:273-95.